Research interests
The science of composite materials is facing a turning point, where the classic paradigm of simple structures comprising high performance materials has reached its limits. Nature drew our attention to complex multi-level structures, which excel in balancing mechanical properties and material resources. The secret lies in the intricate geometrical features found at each structural level, self-assembled from nanoscale, through microscale to macroscale. These materials possess unique properties at nano and micro scales, demonstrated by our investigations of electrospinning of nanofibers.
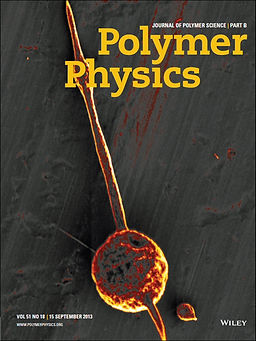
Electrospun PMMA nanofiber with bead
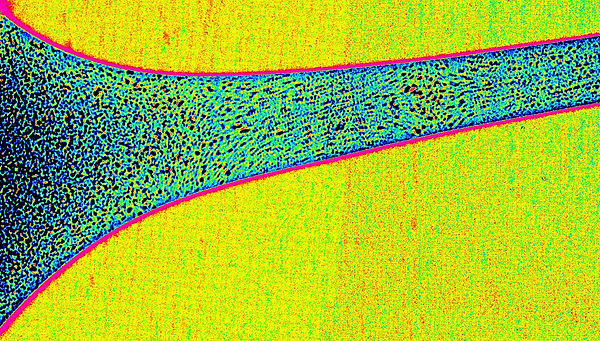
X-ray imaging of an electrospinning jet of PEO polymer solution with silica microbeads
My main research interest is in the effect of internal structural geometry on the mechanical properties of an assembled composite. The physical and mechanical properties of objects at micro and nano scales exhibit size dependence, caused by molecular rearrangment due to confinement, surface tension, and stretching. At the molecular level, I investigated the conformation of polymer chains in electrospun fibers, and showed how flow-induced orientation simultaneously improves stiffness, strength, and toughness by more than an order of magnitude, and how the insertion of nanowires further enhances orientation. At the nano level, I investigated the role of the geometry of nanofillers such as carbon nanotubes, and showed the potential contribution of their hollow shape to mechanical properties. Shape memory polymers are another example for the effect of molecular morphology on macro time-temperature properties.
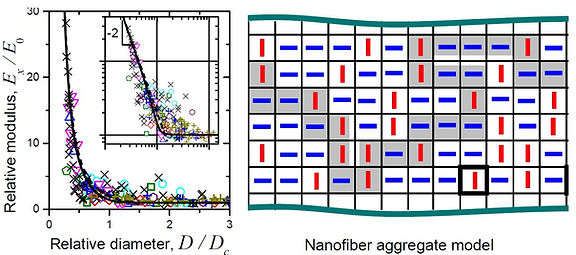
Material stiffness (elastic modulus) increases at small scales as a result of molecular orientation
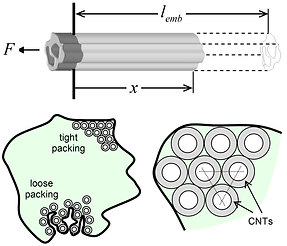
Effect of shape and packing of hollow nano-fillers
Nanofillers currently do not live up to their expected strength because of defects and agglomeration. My analysis of carbon nanotubes with vacancy defects has shown that their toughness conforms to classic fracture mechanics, an important finding in view of the scale involved. I further investigated a multilevel composite reinforced by microscale carbon nanotube fibers, and have shown its potential in self assembly of nanocomponents and in absorbing energy during failure. Another approach was to deposit carbon nanotubes from a solution on fibers to achieve a homogenous scaffold, using Evaporation-driven CNT deposition.
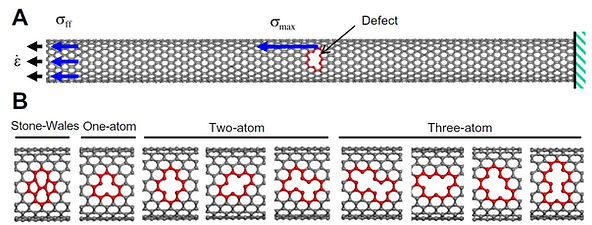
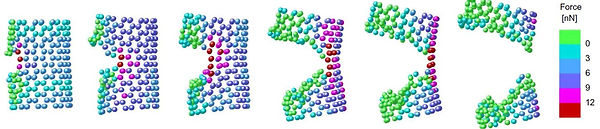
Molecular dynamics simulation of failure of carbon nanotubes (CNTs) with embedded defects

multilevel hierarchical composite consisting of carbon nanotube fibers
We revisited the classic fiber-reinforced composite, and introduced the concept of intermittent fiber beading. I have shown that beaded fibers can potentially improve the gripping of fibers by the matrix, and absorb more energy by plastically displacing the matrix during failure. I completed modeling of the stiffness and strength of such structures, by extending the classic shear-lag theory to beaded fibers. Further research carried out for better theoretical and experimental understanding of the complex toughening mechanisms of beaded fiber composites.
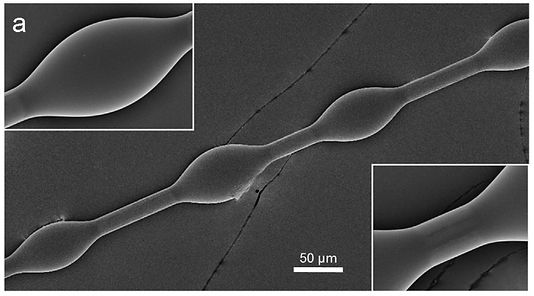

Epoxy beads on glass fibers embedded in a composite enhance strength and toughness

Theoretical and finite-element modeling of beaded fibers stresses
A fascinating phenomenon at small size scales is the transition from brittless to ductility, an important toughening mechanism. The model we are using are epoxy nano and micro fibers. Epoxy is highly brittle and therefore not suitable for structural applications, but our investigation with mechanically drawn and electrospun epoxy fibers indicate that it becomes ductile at these scale.
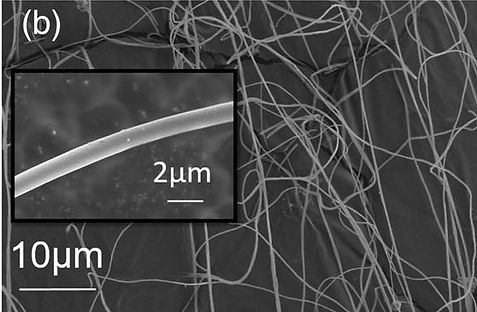
Electrospun epoxy microfibers
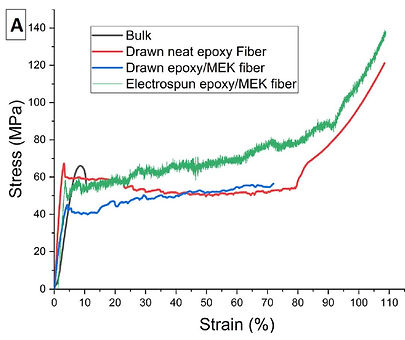
Strength and ductility of epoxy microfibers compared to bulk epoxy
Biology is a never-ending source of inspiration for sructural design at small scales, built bottom-to-top from nanoscale to macroscale. I investigated the micron-size nested helicoids (called Bouligands) in the scorpion cuticle (exoskeleton), and modeled their structural behavior at different hierarchical levels. These investigations revealed the intricate strenghtening and toughening mechanisms of biological structures, such as isotropy, crack diversion, and fracture energy absorption.

Scorpion cuticle intricate micro/nano structure showing tightly-packed helical structures
